Decarbonizing energy in Lithium production.
%20(1).png)
Decarbonizing lithium production with Kraftblock
The stories of white gold are as volatile and reactive as the metal lithium itself. Its presence is found everywhere, from EV batteries to laptops to mobiles. All of this was made possible by a decade-long “lithium rush,” powered by cheap coal and gas. Since the rush is about to escalate further, it is a subject to “GotoZero” campaigns (Grant et al., 2010). Even the recent surge in EV sales (Sun et al., 2022), which drove up the demand and lithium prices, is insufficient to meet lithium extraction plants' sustainability and profitability goals.
Brine mining, the extraction of compounds from a salty solution, and hard rock extraction account for 90% of lithium production. While both are energy-intensive, brine mining is water-intensive and exceptionally harsh on the local environment and communities (Alam et al., 2022). Today, brine mining accounts for 50% of lithium extraction and involves pumping highly saline water and solar baths. While hard rock mining accounts for 40% (Salakjani et al., 2019), it is two times more carbon intensive than brine mining per ton of lithium product produced. Should these methods continue as is, the high CO2 emissions will cut into the desired profit margins of rock mining investments despite demands.
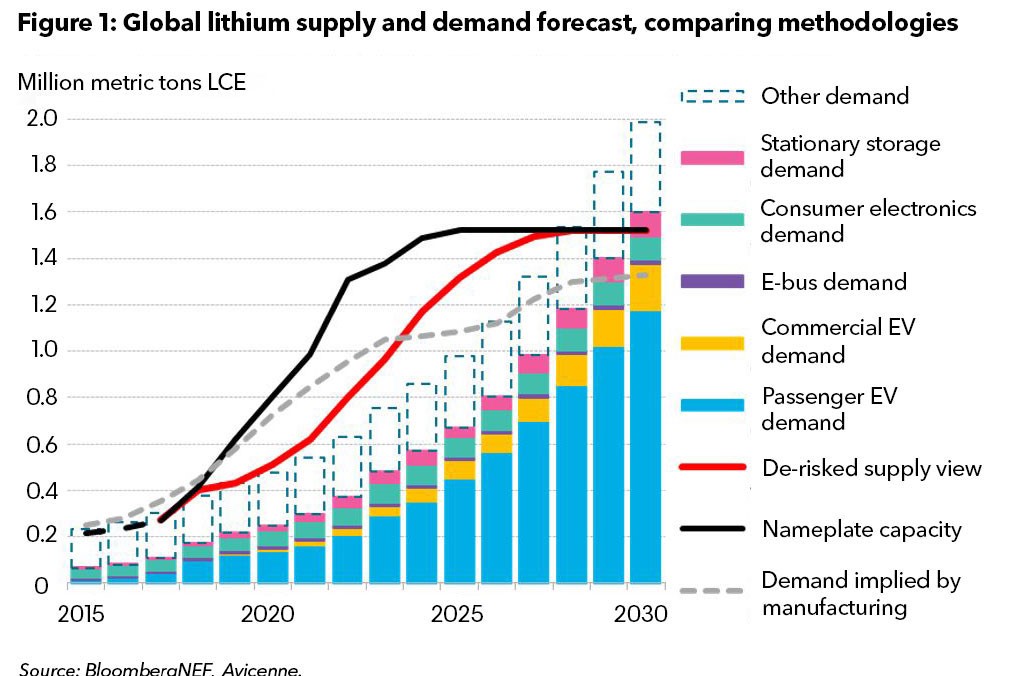
Electrical energy for the breakdown of spodumene, the main source of lithium, heavily depends on burning fossil fuels while roasting spodumene and is a main source of carbon emissions (Grant et al., 2020). This begs the question: Can fossil fuel use be replaced and energy efficiency improved?
If it is, then comminution and calcification are the steps to focus on.
.png)
Grinding out profits and crushing CO2
Spodumene is the main source of lithium in hard rock mining (Salakjani et al., 2019). To release spodumene from pegmatite, the ore is ground and crushed in a process called comminution (Tadesse et al.,2019). Comminution is a key process in metallic mineral extraction from the rock as it prepares the material for sorting.
Using comparable metrics derived from gold, Ballantyne et al. (2012) attributed half a gold mine's total electric usage was used on comminution, from which 95%of energy was lost to heat. As electrical grids power the grinders, the amount of CO2 emissions depends on the source of electricity generated at the site or in the country. Still, three-quarters of grinding energy is lost in heating the slurry to 50 degrees (Napier-Munn, 2015). So, recycling the remaining waste heat (25%) may only lead to minimal overall gains in efficiency.
This only leaves two obvious approaches for lowering CO2 emissions from comminution. The first requires a transition to solar and wind energy to supply the local power grids and, thus, the grinders. This can take decades. Another viable option for extraction plants is to focus on the steps leading up to grinding.
Before grinding, pegmatite can be weakened. Blasting instead of rock-cutting machines creates microfractures (Napier-Munn,2014), weakening the rocks. Thus, the energy requirements to power the grinders are lower. But looking beyond grinding can lead to even more savings.
Replacing fossil fuels and redistributing heat via calcination
Calcination is the process used to turn natural spodumene, as sorted out from the ground pegmatite, into beta-spodumene. Beta-spodumene is more reactive to chemical processes required to extract lithium from the mineral. It involves roasting spodumene which starts at 800°C and ends at 1100°C. This means it can be electrified and fossil fuels can be replaced with Kraftblock’s Net-Zero Heat System, as a direct link to the furnace.
Even when replacing the fossil fuels in the existing kilns, waste heat during roasting is generated. This can be utilized with a heat exchanger and thermal energy storage and released when needed for preheating or other heat demands. Those ‘heat-intensive’ (90 to 250°C) processes can be decarbonized in total or in parts by the utilization of waste heat from calcination. They also can be electrified with renewables and a storage. The processes are for example:
- preheating spodumene before roasting,
- heating of sulfuric acid used in acid leaching / roasting,
- drying of the leachate after acid leaching (crystallization).
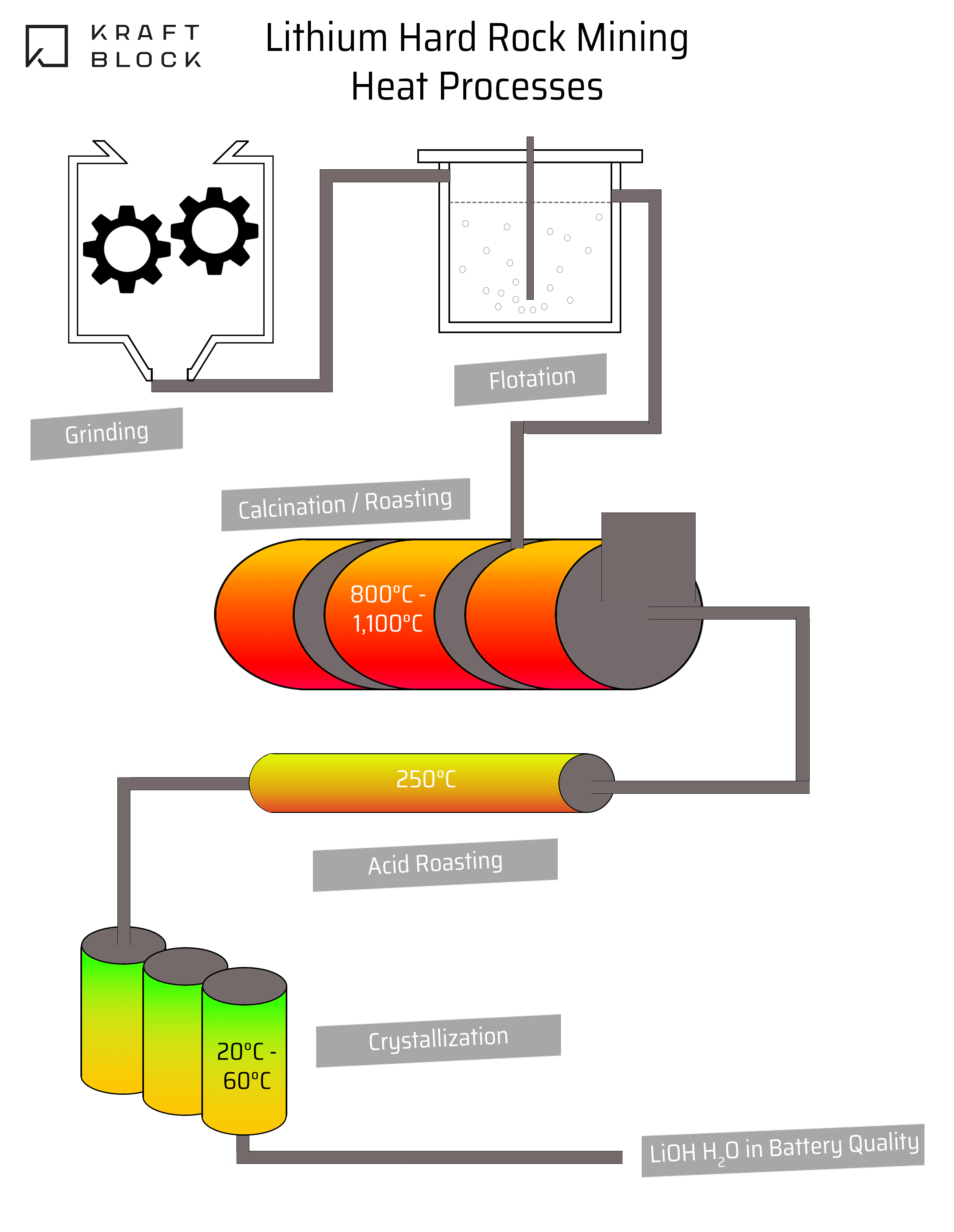
Especially the acid roasting after the calcination process which is at 250°C can be decarbonized by waste heat or electrified. The waste heat from calcination most likely needs to be transferred in a heat exchanger due to the dust contamination.
Wherever high temperatures for metal refinement are needed, a high potential exists for recycling that heat and mitigating carbon emissions (Miró et al., 2016). With mounting pressure to cut CO2 emissions across entire supply chains, keeping hard rock lithium mining energy-efficient will be critical to offset the need and dependency on brine mining.
Thus, electrifying the process with thermal energy storage and reusing other wasted heat to lower fossil fuel emissions could be a game-changer for hard-rock lithium mining until other options for the precious metal become available. But will it?
Will there be other sources or alternatives to lithium?
The increasing demand for net-zero emissions and growing EV sales drive the need to search for portable energy solutions beyond lithium and lithium mining. Battery manufacturing and supply is a clear priority, with the United States funding $1.6 billion for lithium and battery projects in 2022 (Geological Survey, 2024). Among them, funds are flowing to recycle lithium from decommissioned lithium batteries.
How much can recycling lithium from lithium batteries help?
There are not enough batteries being decommissioned today to meet the increasing demands for new battery power (Crownhart, 2023). More than 80 GWh of lithium-capable batteries are set to be decommissioned annually by 2030 (Yin et al., 2020). However, demand for Li-ion batteries is predicted to be 4,700 GWh by 2030 (McKinsey & Company, 2023). Based on these numbers, supply from recycling efforts will contribute 1-2% of future demands as we continually dip into our worldwide reserves. Recycling is a topic still in development. High-temperature processes and pyrometallurgy, for example in the case of spent lithium battery cathodes, appear to be beneficial and could need green process heat (Wang et al., 2024).
%2520(2).png)
Will new battery technologies offset our need for lithium?
A key performance indicator of mass batteries, particularly for transportation use cases, is energy density, watt-hours per kilogram. Future battery technologies that provide similar metrics to Li-ion batteries are also Lithium-based. They are Li-sulfur, Li-air or solid-state with metallic lithium. In short, no near commercial-ready battery alternative can match what lithium provides.
Current research efforts on alternative battery chemistry prioritize proof-of-concept over mass production (Walter etal., 2020). In the meantime, as the global demand for lithium increases, the pressure to adopt a less energy-intensive comminution and calcination process goes unchanged. One of those is adopting commercially deployable strategies available today, such as Kraftblock’s waste heat recycling and energy storage units.
References
Alam, M. A., & Sepúlveda, R. (2022).Environmental degradation through mining for energy resources: The case of theshrinking Laguna Santa Rosa wetland in the Atacama Region of Chile. Energy Geoscience, 3(2), 182-190. https://doi.org/10.1016/j.engeos.2021.11.006
Crownhart, C. (2023, January 4). What’s nextfor batteries in 2023. MIT Technology Review.https://www.technologyreview.com/2023/01/04/1066141/whats-next-for-batteries/amp/
Ballantyne, G. R., & Powell, M. S. (2014).Benchmarking comminution energy consumption for the processing of copper andgold ores. Minerals Engineering, 65(65), 109–114. https://doi.org/10.1016/j.mineng.2014.05.017
Geological Survey. (2024). Mineral commodity summaries 2024. U SGovt. Printing Office.
Grant, A., Deak, D., & Pell, R. (2020).The CO2 Impact of the 2020s Battery Quality Lithium Hydroxide Supply Chain.Minviro reporthttps://static1.squarespace.com/static/5c9aa323c46f6d499a2ac1c5/t/5fe8ae081c123d7f84d3211d/1609084425044/The+CO2+Impact+of+the+2020s+Battery+Quality+Lithium+Hydroxide+Supply+Chain.pdfDate of last access: Feb 21, 2024
Miró, L., Gasia, J., & F. Cabeza, L.(2016). Thermal energy storage (TES) for industrial waste heat (IWH) recovery:A review. Applied Energy, 179(179), 284–301. https://doi.org/10.1016/j.apenergy.2016.06.147
Napier-Munn, T. Is progress inenergy-efficient comminution doomed? Miner. Eng. (2014), http://dx.doi.org/10.1016/ j.mineng.2014.06.009
Salakjani, N.Kh., Singh, P., & Nikoloski, A. N. (2019). Productionof Lithium –A Literature Review. Part 2. Extraction from Spodumene. MineralProcessing and Extractive Metallurgy Review, 1–16. https://doi.org/10.1080/08827508.2019.1700984
Sun, X., Ouyang, M., & Hao, H. (2022). Surging lithiumprices will allow the electric vehicle to boom. Joule, 6(8), 1738-1742.
Tabelin, C. B., Dallas, J., Casanova, S.,Pelech, T., Bournival, G., Saydam, S., & Canbulat, I. (2021). Towards alow-carbon society: A review of lithium resource availability, challenges andinnovations in mining, extraction and recycling, and future perspectives.Minerals Engineering, 163(1), 106743. https://doi.org/10.1016/j.mineng.2020.106743
Tadesse, B., Makuei, F., Albijanic, B., &Dyer, L. (2019). The beneficiation of lithium minerals from hard rock ores: Areview. Minerals Engineering, 131(131), 170–184. https://doi.org/10.1016/j.mineng.2018.11.023
Walter, M., Kovalenko, M. V., & Kravchyk,K. V. (2020). Challenges and benefits of post-lithium-ion batteries. NewJournal of Chemistry, 44(5), 1677–1683. https://doi.org/10.1039/C9NJ05682C
Wang, J., You, X., She, X., Xue. Q. (2024).Research on the process of carbon thermal reduction for recovery andresynthesis of LiNi0.6Co0.2Mn0.2O2. Journalof Material Cycles and Waste Management, 26, 346–359.
https://doi.org/10.1007/s10163-023-01835-0
Yin, J., Pan, M., Chen, Z., & Li, J.(2020). Discussion on the recycling ecological chain and commercial model ofdecommissioned batteries. IOP Conference Series: Materials Science andEngineering, 793(1), 012001–012001. https://doi.org/10.1088/1757-899x/793/1/012001